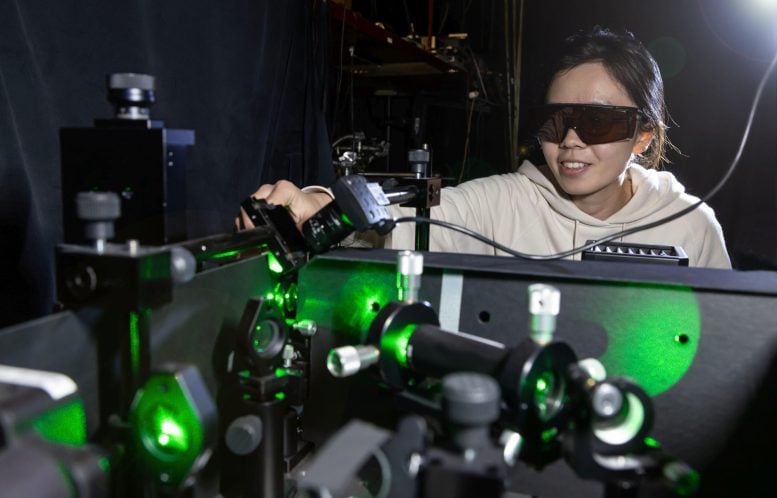
HDR photoluminescence measuring station: Dr. Genghua Yan was responsible for a large part of the measurements. Credit: Forschungszentrum Jülich/Ralf-Uwe Limbach
Researchers at Forschungszentrum Jülich have identified a special form of protection against recombination for free charge carriers in perovskite solar cells, discovered by means of innovative photoluminescence measurements.
Perovskite solar cells, known for their high efficiency and relatively low production cost, have consistently surprised researchers. Recently, scientists at Forschungszentrum Jülich revealed a new aspect of these cells using an innovative photoluminescence measurement method. They discovered that the loss of charge carriers in this type of cell follows different physical laws than those known for most <span class="glossaryLink" aria-describedby="tt" data-cmtooltip="
” data-gt-translate-attributes=”[{"attribute":"data-cmtooltip", "format":"html"}]” tabindex=”0″ role=”link”>semiconductors. This unique behavior could explain the cells’ impressive efficiency. These findings were published in the journal Nature Materials.
Perovskite solar cells are regarded as highly promising for photovoltaics, even if their stability leaves much to be desired. Cells of this type are inexpensive to print and very efficient. In the last decade, their efficiency has doubled to over 25 % and is therefore currently on par with conventional solar cells made of silicon. Further improvements also appear to be possible in the future.
“An important factor here is the question of how long excited charge carriers remain in the material, in other words, their lifetime,” explains Thomas Kirchartz. “Understanding the processes is crucial to further improving the efficiency of perovskite-based solar cells.” The electrical engineer is the head of a working group on organic and hybrid solar cells at Forschungszentrum Jülich’s Institute of Energy and Climate Research (IEK-5).
It’s the lifetime that counts
In a solar cell, electrons are dislodged by photons and raised to a higher energy level from the valence band to the conduction band. Only then can they move more freely and flow through an external circuit. They can only contribute to electrical energy generation if their lifetime is long enough for them to pass through the absorber material to the electrical contact. An excited electron also leaves a hole in the underlying valence band – a mobile vacancy that can be moved through the material like a positive charge carrier.
It is mainly defects in the crystal lattice which ensure that excited electrons quickly fall back down to lower energy levels again. The electrons affected are then no longer able to contribute to the current flow. “This mechanism is also known as recombination and is the main loss process of every solar cell,” says Kirchartz.
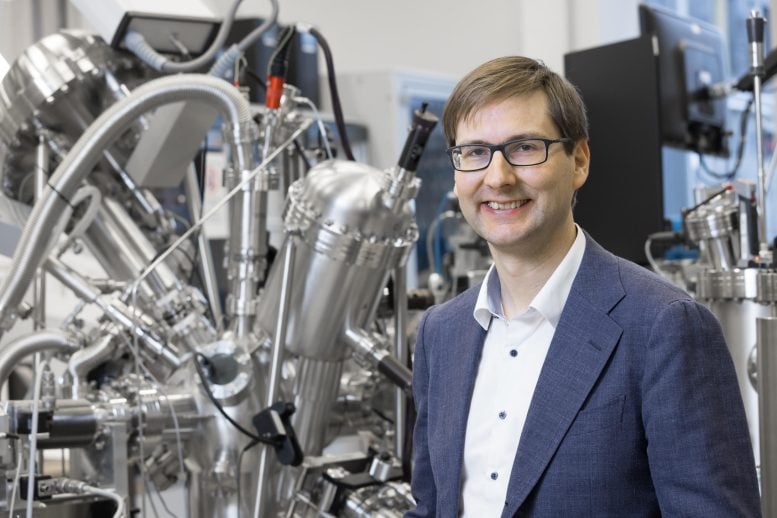
Prof. Thomas Kirchartz is using innovative analytical methods to research new printable solar cells, for example from halide perovskites or molecular semiconductors. Credit: Forschungszentrum Jülich/Ralf-Uwe Limbach
Recombination crucial for efficiency
No solar cell is perfect on an atomic level; each one has different types of defects due to the manufacturing process. These defects or foreign atoms in the lattice structure are the collection points where electrons and holes tend to come together. The electrons then fall back into the valence band and become worthless in terms of electricity generation.
“It had previously been assumed that recombination is predominantly triggered by defects that are energetically located in the middle between the valence and conduction bands. This is because these deep defects are similarly accessible to excited electrons and their counterparts, the holes,” says Kirchartz. Indeed, this is likely true for most types of solar cells.
Shallow defects dominate
However, Kirchartz and his team have now disproved this assumption for perovskite solar cells and shown that the shallow defects are ultimately decisive in terms of their final efficiency. Unlike the deep defects, they are not located in the middle of the band gap, but very close to the valence or conduction band.
“The cause of this unusual behavior has not yet been fully clarified,” Kirchartz adds. “It is reasonable to assume that deep defects simply cannot exist in these materials. This restriction may also be one of the reasons for the particularly high efficiency of the cells.”
New HDR measurement technique with extended dynamic range
The observation was only made possible by innovative transient photoluminescence measurements. In previous measurements, it was not possible to distinguish loss processes caused by shallow defects from those caused by other factors.
The new measuring method developed by Thomas Kirchartz and his team at Forschungszentrum Jülich delivers data with a significantly increased dynamic range compared to conventional technology, i.e. data over a larger measuring range and with better fine gradation. The process is based on a similar principle to HDR images in high dynamic range quality. The dynamic range of the camera is increased by superimposing different images or measurements – in this case, signals with different levels of amplification – to create a data set.
Reference: “Shallow defects and variable photoluminescence decay times up to 280 µs in triple-cation perovskites” by Ye Yuan, Genghua Yan, Chris Dreessen, Toby Rudolph, Markus Hülsbeck, Benjamin Klingebiel, Jiajiu Ye, Uwe Rau and Thomas Kirchartz, 9 January 2024, Nature Materials.
DOI: 10.1038/s41563-023-01771-2