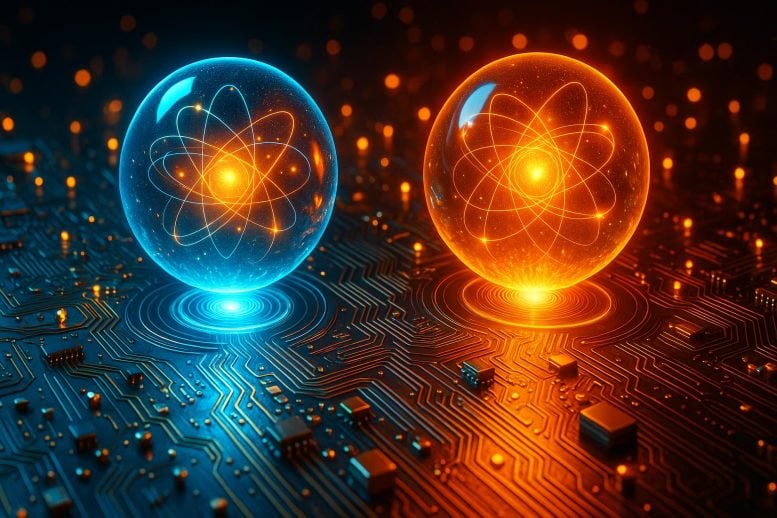
A new MIT-designed circuit achieves record-setting nonlinear coupling, allowing quantum operations to occur dramatically faster.
The heart of this advance is the “quarton coupler,” which boosts both light-matter and matter-matter interactions. This progress could lead to quicker quantum readouts, crucial for error correction and computation fidelity.
Unlocking Quantum Computing’s Speed Potential
Quantum computers have the potential to revolutionize fields like materials science and <span class="glossaryLink" aria-describedby="tt" data-cmtooltip="
” data-gt-translate-attributes=”[{"attribute":"data-cmtooltip", "format":"html"}]” tabindex=”0″ role=”link”>artificial intelligence. They could one day simulate complex materials or accelerate <span class="glossaryLink" aria-describedby="tt" data-cmtooltip="
” data-gt-translate-attributes=”[{"attribute":"data-cmtooltip", "format":"html"}]” tabindex=”0″ role=”link”>machine learning models far beyond today’s capabilities.
However, these breakthroughs will only be possible if quantum computers can operate extremely quickly. To function reliably, they must measure quantum states and apply error corrections before accumulating errors compromise the results. This process, called readout, depends on how strongly photons (light particles carrying quantum information) interact with artificial atoms—quantum components that store this information.
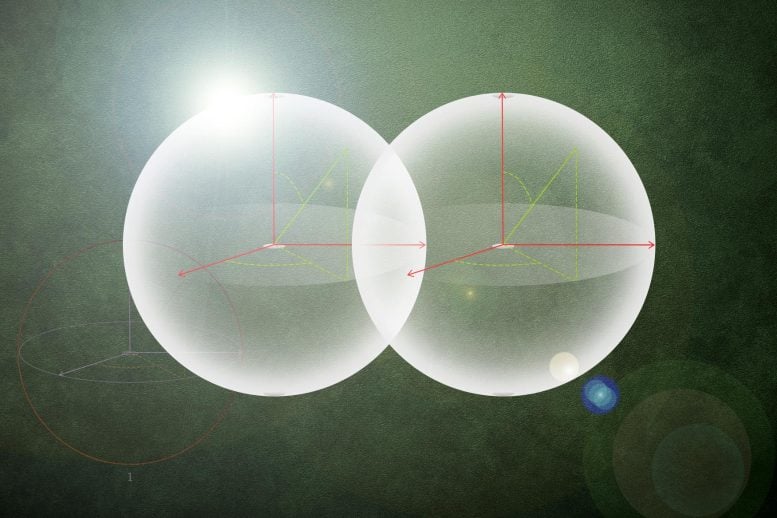
Achieving Stronger Light-Matter Coupling
<span class="glossaryLink" aria-describedby="tt" data-cmtooltip="
” data-gt-translate-attributes=”[{"attribute":"data-cmtooltip", "format":"html"}]” tabindex=”0″ role=”link”>MIT researchers have now demonstrated what may be the strongest nonlinear light-matter coupling ever observed in a quantum system. This breakthrough could allow quantum computers to perform operations and readouts in just a few nanoseconds.
Using a novel superconducting circuit design, the team achieved coupling strength roughly 10 times greater than previous efforts. Such a boost could significantly increase the speed of quantum processors.
While this architecture isn’t ready for deployment in practical quantum computers, proving the underlying physics is a crucial milestone, says lead author Yufeng “Bright” Ye, SM ’20, PhD ’24.
“This would really eliminate one of the bottlenecks in <span class="glossaryLink" aria-describedby="tt" data-cmtooltip="
” data-gt-translate-attributes=”[{"attribute":"data-cmtooltip", "format":"html"}]” tabindex=”0″ role=”link”>quantum computing. Usually, you have to measure the results of your computations in between rounds of error correction. This could accelerate how quickly we can reach the fault-tolerant quantum computing stage and be able to get real-world applications and value out of our quantum computers,” says Ye.
He is joined on the paper by senior author Kevin O’Brien, an associate professor and principal investigator in the Research Laboratory of Electronics at MIT who leads the Quantum Coherent Electronics Group in the Department of Electrical Engineering and Computer Science (EECS), as well as others at MIT, MIT Lincoln Laboratory, and Harvard University. The research was published on April 30 in <span class="glossaryLink" aria-describedby="tt" data-cmtooltip="
” data-gt-translate-attributes=”[{"attribute":"data-cmtooltip", "format":"html"}]” tabindex=”0″ role=”link”>Nature Communications.
The Quarton Coupler Breakthrough
This physical demonstration builds on years of theoretical research in the O’Brien group.
After Ye joined the lab as a PhD student in 2019, he began developing a specialized <span class="glossaryLink" aria-describedby="tt" data-cmtooltip="
” data-gt-translate-attributes=”[{"attribute":"data-cmtooltip", "format":"html"}]” tabindex=”0″ role=”link”>photon detector to enhance quantum information processing.
Through that work, he invented a new type of quantum coupler, which is a device that facilitates interactions between qubits. Qubits are the building blocks of a quantum computer. This so-called quarton coupler had so many potential applications in quantum operations and readout that it quickly became a focus of the lab.
Nonlinearity and Quantum Speed
This quarton coupler is a special type of superconducting circuit that has the potential to generate extremely strong nonlinear coupling, which is essential for running most quantum algorithms. As the researchers feed more current into the coupler, it creates an even stronger nonlinear interaction. In this sense, nonlinearity means a system behaves in a way that is greater than the sum of its parts, exhibiting more complex properties.
“Most of the useful interactions in quantum computing come from nonlinear coupling of light and matter. If you can get a more versatile range of different types of coupling, and increase the coupling strength, then you can essentially increase the processing speed of the quantum computer,” Ye explains.
Measuring Quantum States Faster
For quantum readout, researchers shine microwave light onto a qubit and then, depending on whether that qubit is in state 0 or 1, there is a frequency shift on its associated readout resonator. They measure this shift to determine the qubit’s state.
Nonlinear light-matter coupling between the qubit and resonator enables this measurement process.
The MIT researchers designed an architecture with a quarton coupler connected to two superconducting qubits on a chip. They turn one qubit into a resonator and use the other qubit as an artificial <span class="glossaryLink" aria-describedby="tt" data-cmtooltip="
” data-gt-translate-attributes=”[{"attribute":"data-cmtooltip", "format":"html"}]” tabindex=”0″ role=”link”>atom, which stores quantum information. This information is transferred in the form of microwave light particles called photons.
“The interaction between these superconducting artificial atoms and the microwave light that routes the signal is basically how an entire superconducting quantum computer is built,” Ye explains.
Building the Fastest Readout Yet
The quarton coupler creates nonlinear light-matter coupling between the qubit and resonator that’s about an order of magnitude stronger than researchers had achieved before. This could enable a quantum system with lightning-fast readout.
“This work is not the end of the story. This is the fundamental physics demonstration, but there is work going on in the group now to realize really fast readout,” O’Brien says.
That would involve adding additional electronic components, such as filters, to produce a readout circuit that could be incorporated into a larger quantum system.
The researchers also demonstrated extremely strong matter-matter coupling, another type of qubit interaction that is important for quantum operations. This is another area they plan to explore with future work.
Toward a Fault-Tolerant Quantum Future
Fast operations and readout are especially important for quantum computers because qubits have finite lifespans, a concept known as coherence time.
Stronger nonlinear coupling enables a quantum processor to run faster and with lower error, so the qubits can perform more operations in the same amount of time. This means the qubits can run more rounds of error correction during their lifespans.
“The more runs of error correction you can get in, the lower the error will be in the results,” Ye says.
In the long run, this work could help scientists build a fault-tolerant quantum computer, which is essential for practical, large-scale quantum computation.
This research was supported, in part, by the Army Research Office, the AWS Center for Quantum Computing, and the MIT Center for Quantum Engineering.
Reference: “Near-ultrastrong nonlinear light-matter coupling in superconducting circuits” by Yufeng Ye, Jeremy B. Kline, Alec Yen, Gregory Cunningham, Max Tan, Alicia Zang, Michael Gingras, Bethany M. Niedzielski, Hannah Stickler, Kyle Serniak, Mollie E. Schwartz and Kevin P. O’Brien, 30 April 2025, Nature Communications.
DOI: 10.1038/s41467-025-59152-z
Never miss a breakthrough: Join the SciTechDaily newsletter.