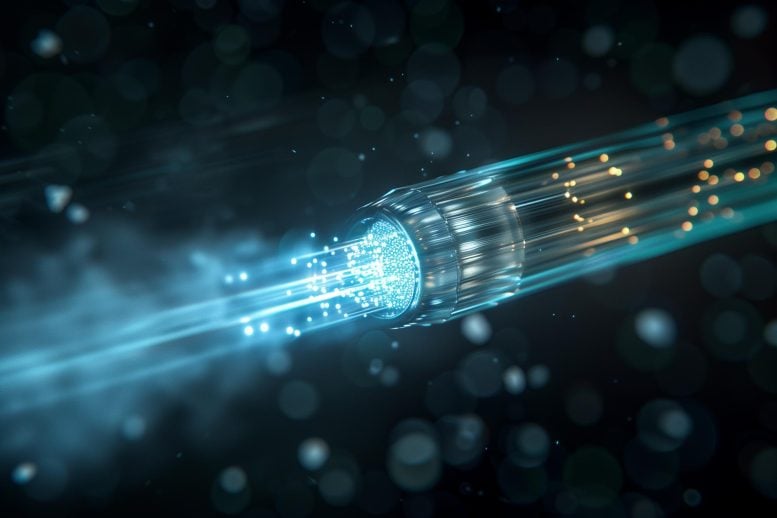
Quantum computing takes a leap forward with fiber-optic-connected qubits, making large-scale and networked quantum systems more feasible.
Qubits—the building blocks of quantum computing—are driving advancements across the tech industry. Among them, superconducting qubits hold great promise for large-scale quantum computers. However, they rely on electrical signals, making them challenging to scale.
In a breakthrough, physicists at the Institute of Science and Technology Austria (ISTA) have successfully developed a fully optical readout for superconducting qubits, overcoming a key technological hurdle. Their findings, recently published in <span class="glossaryLink" aria-describedby="tt" data-cmtooltip="
” data-gt-translate-attributes=”[{"attribute":"data-cmtooltip", "format":"html"}]” tabindex=”0″ role=”link”>Nature Physics, could pave the way for more scalable and efficient <span class="glossaryLink" aria-describedby="tt" data-cmtooltip="
” data-gt-translate-attributes=”[{"attribute":"data-cmtooltip", "format":"html"}]” tabindex=”0″ role=”link”>quantum computing systems.
The Race for Scalable Quantum Computers Continues
After a year-long surge, quantum computing stocks came to a sudden halt just days into the International Year of Quantum Science and Technology. The slowdown was triggered by Nvidia CEO Jensen Huang’s keynote at CES 2025, where he predicted that “very useful quantum computers” were still two decades away.
Despite market fluctuations and industry skepticism, the race to build scalable quantum computers remains intense. These machines have the potential to outperform classical computers in specific calculations, but significant technical challenges must be addressed before they become truly practical.
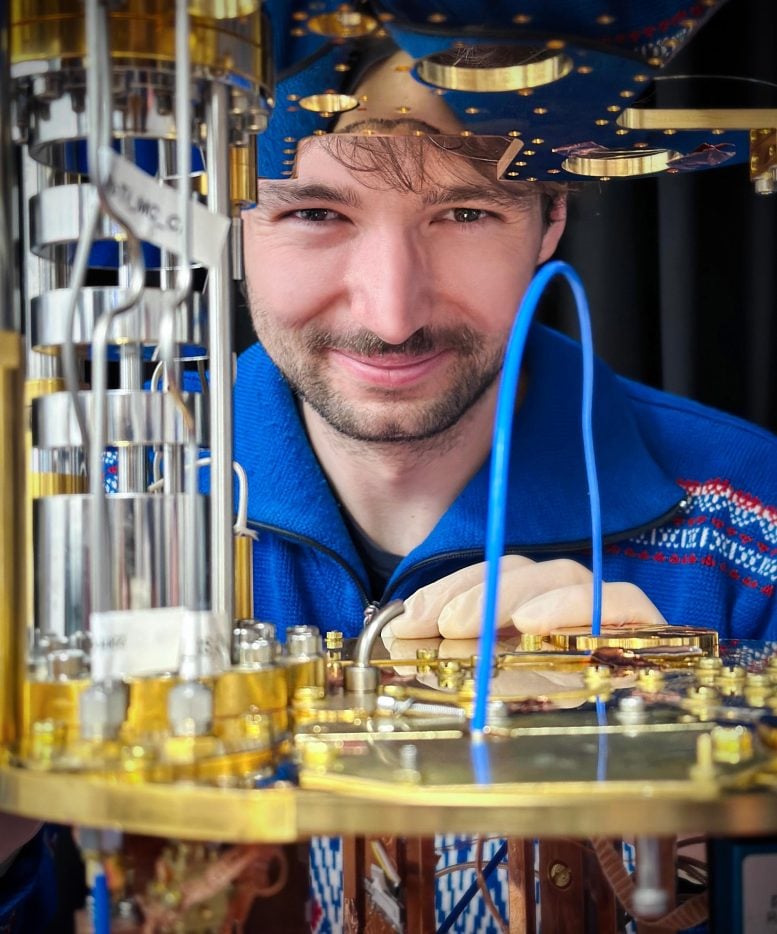
A Breakthrough in Quantum Hardware Scaling
Now, a team of physicists from Professor Johannes Fink’s group at the Institute of Science and Technology Austria (ISTA) has taken a critical step toward making quantum computing more viable. They developed a method that allows qubits to communicate using fiber optics, significantly reducing the need for bulky cryogenic hardware. “This new approach might allow us to increase the number of qubits so they become useful for computation.,” explains Georg Arnold, a co-first author and former PhD student in the Fink group. “It also lays the foundation for building a network of superconducting quantum computers connected via optical fibers at room temperature.”
The Challenges of Applying Fiber Optics to Quantum Systems
While fiberoptics have revolutionized the telecom industry with their multiple advantages over electrical transmission and enabled high-speed communication, applying optics to quantum hardware is no easy task. Superconducting quantum computers, which use special physical properties of materials at temperatures near <span class="glossaryLink" aria-describedby="tt" data-cmtooltip="
” data-gt-translate-attributes=”[{"attribute":"data-cmtooltip", "format":"html"}]” tabindex=”0″ role=”link”>absolute zero, present a challenge of their own.
To realize superconducting qubits, tiny electrical circuits are cooled to extremely low temperatures where they lose all electrical resistance and can thus maintain a flowing current indefinitely. “Thus, superconducting qubits are electrical by definition. To make them, we must reach temperatures of only a few thousandths of a degree above absolute zero. That’s even colder than space,” says Arnold.
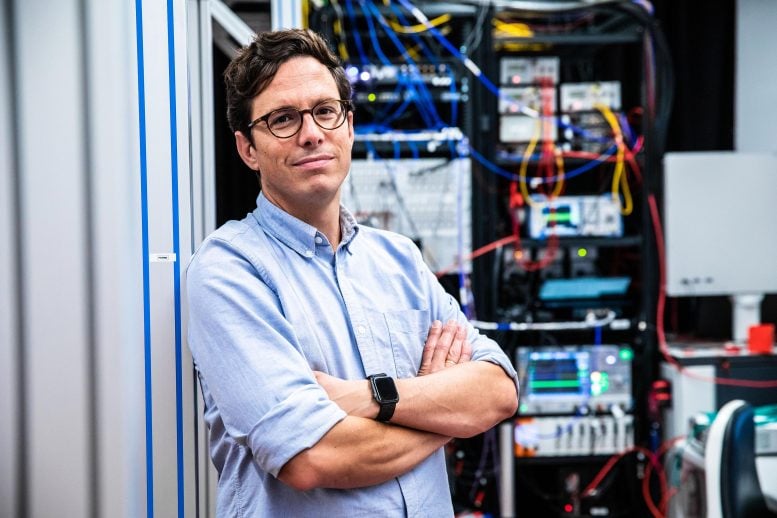
Superconducting Qubits and Their Cold Reality
However, electrical signals have a comparably low bandwidth, meaning they transmit little information per unit of time. Easily overwhelmed by noise, they are also prone to information loss. Also, the required wiring dissipates lots of heat. Thus, the “qubit readout”, i.e., detecting and measuring qubits by sending an electric signal that they reflect, requires colossal cryogenic cooling as well as elaborate and expensive electrical components for filtering and amplification.
On the other hand, higher-energy optical signals—for example, at telecom wavelengths—propagate in thin optical fibers with minuscule losses. In addition, they have a considerably lower heat dissipation and much higher bandwidth. So, using them to push the limits of superconducting quantum hardware would be ideal, if only the qubits would understand their language.
‘Translating’ Optical Signals for Qubits
To achieve a fully optical readout in superconducting quantum hardware, the team needed to find a way to ‘translate’ the optical signal to the qubits and back.
“Ideally, one would try to get rid of all electrical signals, as the required wiring transports a lot of heat into the cooling chambers where the qubits are. But this is not possible,” says co-first author Thomas Werner, a PhD student in the Fink group at ISTA.
So, the researchers thought of using an electro-optic transducer to convert the optical signal to a microwave frequency—an electrical signal that the qubits can understand. In response, the qubits reflect a microwave signal that the transducer converts to optics.
Werner highlights the delicacy of the task, “We showed that we can send infrared light close to the qubits without making them lose their superconductivity.” Using the electro-optic transducer as a switch allowed the team to connect the qubits directly to the outside world.
Breaking the Qubit Barrier: A Scalable Future
To do ‘useful’ computation with quantum computers, thousands or even millions of qubits are necessary. However, the infrastructure has difficulty keeping up because the cryogenic cooling requirements to detect and measure them are prohibitive. “Our technology can decrease the heat load of measuring superconductive qubits considerably. This will allow us to break the qubit barrier and scale up the number of qubits that can be used in quantum computing,” says Arnold.
Achieving a fully optical readout of superconducting qubits also allowed the researchers to rid the setup of many of its cumbersome electrical components. The electrical signal in conventional readout systems is highly error-prone, requiring large-scale signal correction using many technically limiting and expensive electrical components that must also be cooled to cryogenic temperatures. “So, by using the electro-optic transducer to disconnect the qubits from the electrical infrastructure, we were able to replace all the remaining parts of the setup with optics,” says Werner. This makes the system not only more robust and efficient, but also reduces its costs.
Connecting Quantum Computers with Light
This technology could help increase the number of usable superconducting qubits even further by allowing scientists to interface multiple quantum computers using light. Currently, quantum computers need so-called “dilution refrigerators” to provide cooling for the entire measurement setup, including any required connections between processor modules.
“But these dilution refrigerators also have practical limitations and can’t be made infinitely large,” says Arnold. In turn, the space and cooling restrictions limit the number of usable qubits. But now, connecting two qubits in two separate dilution refrigerators using an optical fiber might be within reach, according to the researchers. “The infrastructure is available, and now we have the technology that allows us to build the first simple quantum computing networks,” says Arnold.
A Step Toward Quantum Networks
The ISTA physicists have reached a significant milestone in developing superconducting quantum hardware, but much more remains to be done. “The performance of our prototype is still quite limited—in particular with regard to the amount of optical power needed and dissipated. Nevertheless, it serves as a proof of principle that a fully optical readout of superconducting qubits is even possible. It will be the industry’s role to push the technique further.”
Reference: “All-optical superconducting qubit readout” 11 February 2025, Nature Physics.
DOI: 10.1038/s41567-024-02741-4
Funding: European Research Council, Horizon 2020 Framework Programme, Austrian Science Fund, ÖAW