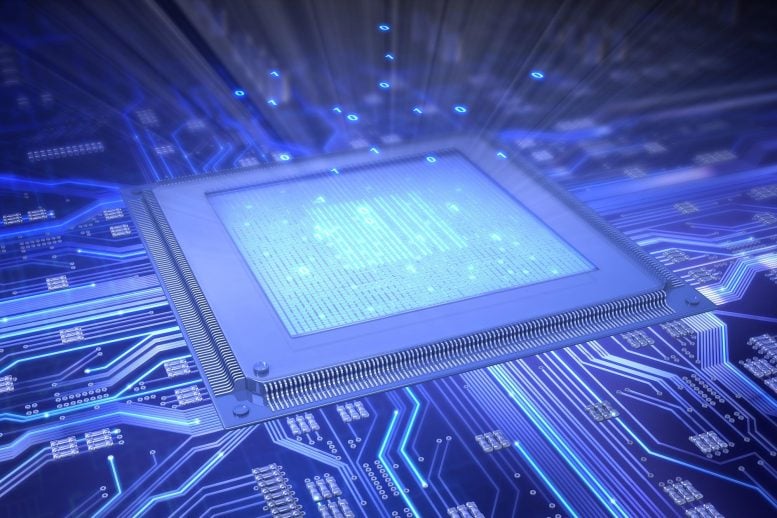
The approach uses lasers and holograms to detect misalignments as small as 0.017 nanometers.
Researchers at the University of Massachusetts Amherst have developed a new method for aligning 3D semiconductor chips by shining a laser through concentric metalenses patterned onto the chips, creating a hologram. Their work, published in <span class="glossaryLink" aria-describedby="tt" data-cmtooltip="
” data-gt-translate-attributes=”[{"attribute":"data-cmtooltip", "format":"html"}]” tabindex=”0″ role=”link”>Nature Communications, could significantly reduce the cost of manufacturing 2D chips, support the development of 3D photonic and electronic chips, and open the door to affordable, compact sensor technologies.
Semiconductor chips power electronic devices by enabling them to process, store, and transmit information. These functions rely on precise patterns of components embedded in the chip. However, the traditional 2D chip design has reached the limits of its technological potential, and 3D integration is now seen as the most promising path forward.
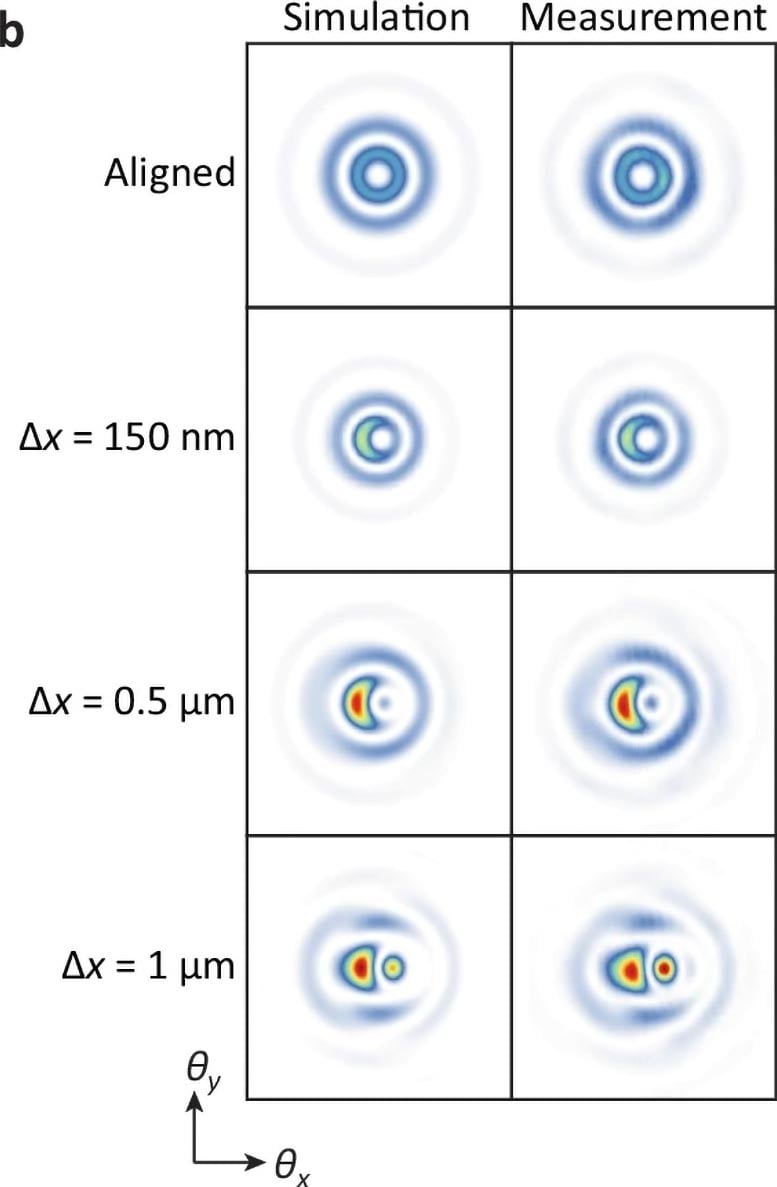
To build a 3D chip, several 2D chips are stacked together. Their layers must be aligned with extreme precision, down to tens of nanometers. This alignment must occur across all three dimensions: front to back, side to side, and the vertical distance between layers (known as the x, y, and z axes).
Limitations of traditional alignment
“The traditional approach for aligning two layers is to look with a microscope for marks (typically corners or crosshairs) on the two layers and to try to overlap them,” explains Amir Arbabi, associate professor of electrical and computer engineering at UMass Amherst and senior author on the paper.
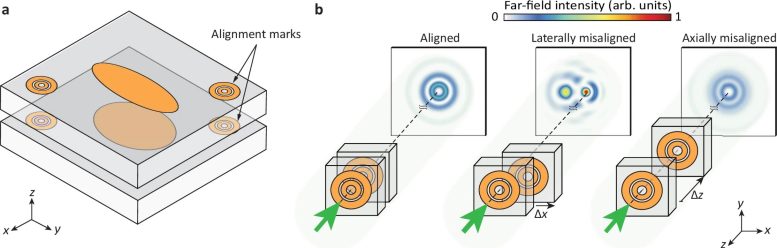
Existing microscope-based alignment methods are ill-suited for making these 3D chips.
“The microscope cannot simultaneously see both crosshairs in focus because the gap between the layers is hundreds of microns, and the motion to refocus between la yers introduces opportunities for the chips to shift and further misalign.” says Maryam Ghahremani, doctoral candidate and lead author on the paper. Also “the smallest features you can resolve are set by the diffraction limit, which is around 200 nanometers,” she adds.
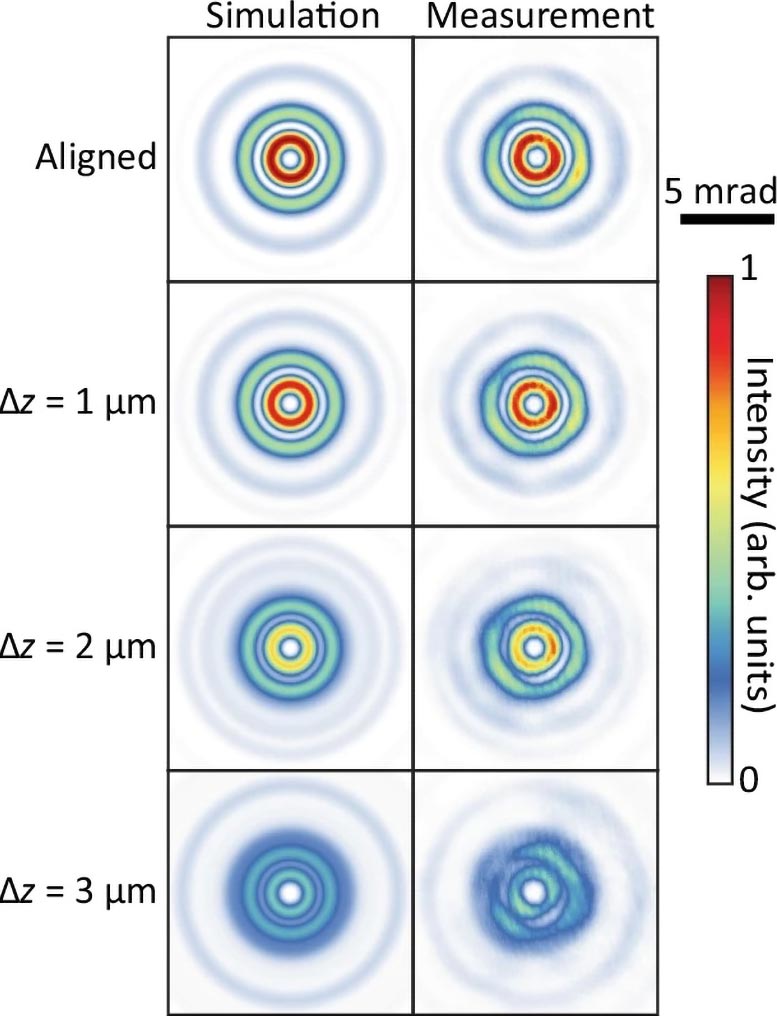
Breakthrough in nanometer-scale detection
The new alignment method created by Arbabi and his team has no moving parts and can see misalignments between two distant layers at a much smaller scale. The researchers were hoping to reach 100-nm precision. Instead, their method finds errors up to 0.017 nm along side-to-side measures (x and y axes) and 0.134 nm when assessing the distance between the two chips (z-axis).
“Consider you have two objects. By looking at the light that goes through them, we can see if one moved by the size of an <span class="glossaryLink" aria-describedby="tt" data-cmtooltip="
” data-gt-translate-attributes=”[{"attribute":"data-cmtooltip", "format":"html"}]” tabindex=”0″ role=”link”>atom with respect to the other one,” Arbabi says, far exceeding their expectations. The naked eye can spot errors as small as a few nanometers, and computers can read even smaller ones.
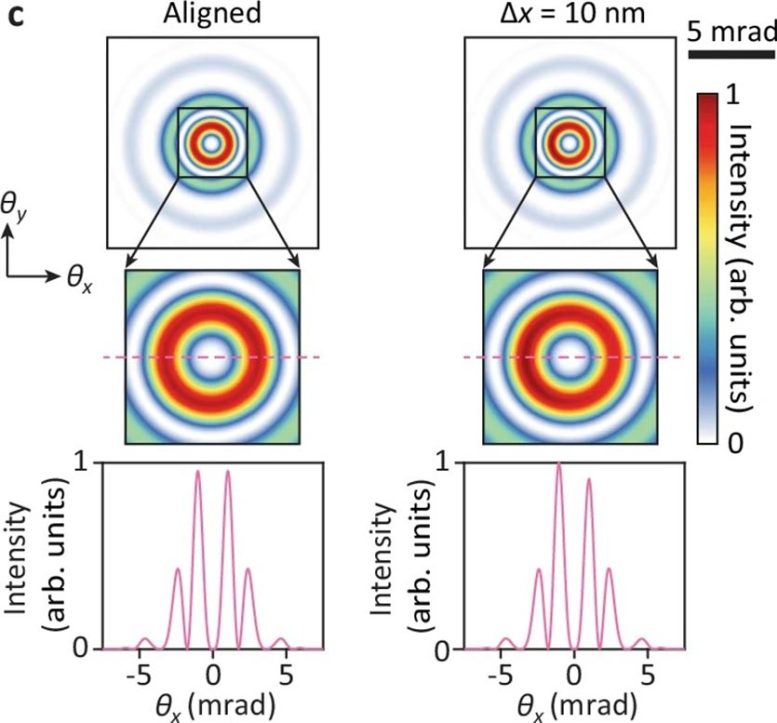
To achieve this, they embedded alignment marks made from concentric metalenses on the semiconductor chip. When light from a laser shines through these marks on both chips, it projects two interfering holograms. “This interference image shows if the chips are aligned or not, as well as the direction and the amount of their misalignment,” says Ghahremani.
“[Chip alignment] is a big, costly challenge for some of the companies that work in manufacturing semiconductor tools,” Arbabi says. “Our approach addresses one of the challenges of making them.” Lower costs also increase access to this technology for smaller startup companies looking to innovate with <span class="glossaryLink" aria-describedby="tt" data-cmtooltip="
” data-gt-translate-attributes=”[{"attribute":"data-cmtooltip", "format":"html"}]” tabindex=”0″ role=”link”>semiconductors.
Arbabi also points out that this method can be used to make displacement sensors that can be used for measuring displacements and other quantities. “Many physical quantities that you want to detect can be translated to displacements, and the only thing you need is a simple laser and a camera,” he says. For instance, “if you want a pressure sensor, you could measure the movement of a membrane.” Anything that involves movement —vibration, heat, acceleration — can in theory be tracked by this method.
Reference: “3D alignment of distant patterns with deep-subwavelength precision using metasurfaces” by Maryam Ghahremani, Andrew McClung, Babak Mirzapourbeinekalaye and Amir Arbabi, 14 October 2024, Nature Communications.
DOI: 10.1038/s41467-024-53219-z
Funding: U.S. National Science Foundation