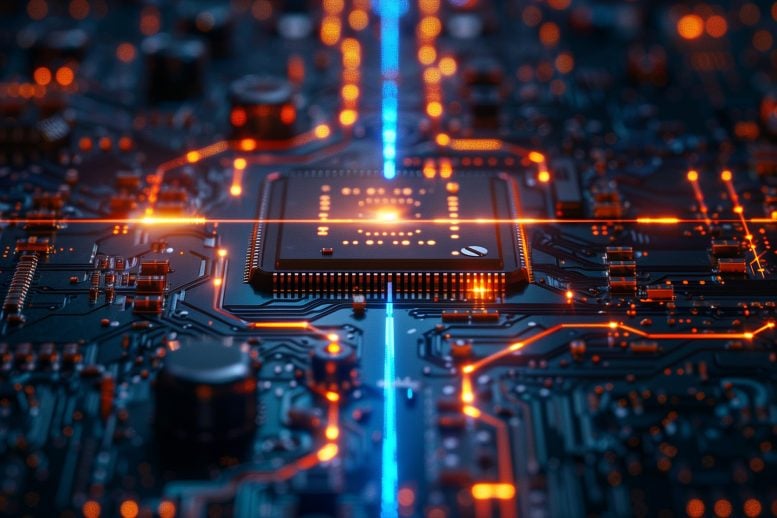
A major breakthrough at POSTECH could dramatically boost AI speeds and device efficiency.
Researchers have, for the first time, decoded how Electrochemical Random-Access Memory (ECRAM) works, using a special technique to observe internal electron behavior even at extreme temperatures. This hidden mechanism, where oxygen vacancies act like shortcuts for electrons, could unlock faster AI systems and longer-lasting smartphones, laptops, and tablets.
Breakthrough at POSTECH: Boosting AI Efficiency
As <span class="glossaryLink" aria-describedby="tt" data-cmtooltip="
” data-gt-translate-attributes=”[{"attribute":"data-cmtooltip", "format":"html"}]” tabindex=”0″ role=”link”>artificial intelligence (AI) continues to evolve, researchers at POSTECH (Pohang University of Science and Technology) have made a breakthrough that could significantly boost the speed and efficiency of AI technologies.
Professor Seyoung Kim and Dr. Hyunjeong Kwak from POSTECH’s Departments of Materials Science & Engineering and Semiconductor Engineering, working alongside Dr. Oki Gunawan from the IBM T.J. Watson Research Center, have become the first to reveal the hidden operating mechanisms of Electrochemical Random-Access Memory (ECRAM[1]). ECRAM is considered a promising next-generation technology for advancing AI. Their groundbreaking findings were recently published in the prestigious journal <span class="glossaryLink" aria-describedby="tt" data-cmtooltip="
” data-gt-translate-attributes=”[{"attribute":"data-cmtooltip", "format":"html"}]” tabindex=”0″ role=”link”>Nature Communications.
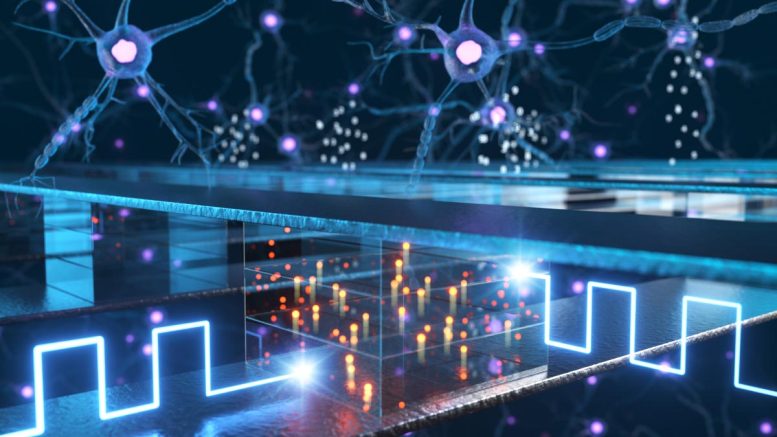
Uncovering ECRAM’s Hidden Mechanisms
As AI capabilities grow, so do the demands for faster and more efficient data processing. Traditional computing architectures separate data storage (“memory”) from data processing (“processors”), which leads to major inefficiencies, requiring constant data transfer between the two units and consuming both time and energy. To solve this problem, researchers have been exploring a new approach called ‘In-Memory Computing.’
‘In-Memory Computing’ enables calculations directly within memory, eliminating data movement and achieving faster, more efficient operations. ECRAM is a critical technology for implementing this concept. ECRAM devices store and process information using ionic movements, allowing for continuous analog-type data storage. However, understanding their complex structure and high-resistive oxide materials has remained challenging, significantly hindering commercialization.
ECRAM: Powering In-Memory Computing
To address this, the research team developed a multi-terminal structured ECRAM device using tungsten oxide and applied the ‘Parallel Dipole Line Hall System’[2]), enabling observation of internal electron dynamics from ultra-low temperatures (-223°C, 50K) to room temperature (300K).
They observed, for the first time, that oxygen vacancies inside the ECRAM create shallow donor states (~0.1 eV), effectively forming ‘shortcuts’ through which electrons move freely. Rather than simply increasing electron quantity, the ECRAM inherently creates an environment facilitating easier electron transport. Crucially, this mechanism remained stable even at extremely low temperatures, demonstrating the robustness and durability of the ECRAM device.
Revealing Electron Shortcuts at Ultra-Low Temperatures
Prof. Seyoung Kim from POSTECH emphasized, “This research is significant as it experimentally clarified the switching mechanism of ECRAM across various temperatures. Commercializing this technology could lead to faster AI performance and extended battery life in devices such as smartphones, tablets, and laptops.”
Notes
- ECRAM (Electrochemical Random-Access Memory): An electrochemical memory device whose channel conductivity varies according to the concentration of ions within the channel. This behavior allows for the expression of analog memory states. The device features a three-terminal structure consisting of a source, drain, and gate. By applying voltage to the gate, ion movement is controlled, and the channel conductivity is read through the source and drain.
- Parallel Dipole Line Hall System, PDL Hall System: A Hall measurement system composed of two cylindrical dipole magnets. When one magnet is rotated, the other rotates automatically, enabling the generation of a strong, superimposed magnetic field. This configuration allows for enhanced sensitivity in observing internal electron behaviors.
Reference: “Unveiling ECRAM switching mechanisms using variable temperature Hall measurements for accelerated AI computation” by Hyunjeong Kwak, Junyoung Choi, Seungmin Han, Eun Ho Kim, Chaeyoun Kim, Paul Solomon, Junyong Lee, Doyoon Kim, Byungha Shin, Donghwa Lee, Oki Gunawan and Seyoung Kim, 19 March 2025, Nature Communications.
DOI: 10.1038/s41467-025-58004-0
This work was supported by K-CHIPS(Korea Collaborative & High-tech Initiative for Prospective Semiconductor Research)funded by the Ministry of Trade, Industry & Energy of Korea (MOTIE).